Click here to view PDF
Grape Seed Extract Laboratory Guidance Document By Steve A. Kupina,a Mark
A. Kelm, PhD,a Maria J. Monagas, PhD,b and Stefan Gafner,
PhDc
aPolyphenolics, Madera, CA 93637 bUnited States
Pharmacopeia, Rockville, MD 20852 bAmerican Botanical Council, Austin, TX 78723 Correspondence: email
Keywords:
Vitis
vinifera, grape seed
extract, adulterant, adulteration Citation (JAMA) style: Kupina SA, Kelm MA, Managas MJ, Gafner S. Grape seed extract laboratory guidance document. Austin, TX: ABC-AHP-NCNPR Botanical Adulterants Prevention Program. 2019.
CONTENTS
1. Purpose
Grape
Seed Extract (GSE)* has
received acceptance almost globally as an ingredient for human consumption. It
is one of the more widely used botanical extracts, due to increasing scientific
findings supporting health benefits.1,2 However, it remains a specialty item
relative to global commodities. In the United States, GSE has ranked among the
top 20 best-selling dietary supplements in the Food, Drug and Mass Market
channel. The motivation behind purposeful
adulteration in commercial products is financial gain (also known as economically
motivated adulteration) and to increase the concentration in proanthocyanidins
(PACs) as primary marker compounds as a means to contribute to the misperception of quality. Adulterants
include other PAC-rich materials (Table 1), which are available at lower cost. Thus,
a bulk distributor of GSE or another manufacturer along the value chain can
take advantage of the chemical similarity between GSE and peanut skin extract
since the spectrophotometric assays typically used in
industry are not specific enough to discriminate between grape seed PACs and
PACs from other plant extracts. Due to reliance on non-specific proximate
assays across the value-chain, adulteration can go undetected downstream in the
commodity chain, such as those involved in distribution, packaging, wholesale,
and retail sales. This laboratory guidance document presents a review of
the various analytical technologies and methods used to differentiate between
grape seed extracts and potential adulterants.
2. Scope
The analysis
of PACs by chromatographic methods such as high-performance
liquid chromatography (HPLC) or high-performance thin-layer chromatography
(HPTLC), which are commonly used to determine the identity of botanical
ingredients, is challenging as current stationary phases have limited capacity
to separate the PACs due to the structural similarity of the many PACs in GSEs
and their polymeric structure. Other tools to authenticate GSEs are
available, but instrumentation to perform the analysis may not be available in
many quality control laboratories or the expertise to perform the necessary
work may be lacking.
This
laboratory guidance document intends to assess analytical methods for GSE
analysis, and to determine the suitability of each of these methods with
regards to its ability to authenticate GSEs and to detect adulteration with
PAC-rich extracts from other plant sources. In addition, existing methods are
evaluated for their ease-of-use in a quality control laboratory. A specific
method for testing GSEs in this Laboratory Guidance Document does not remove
the responsibility of quality control and laboratory personnel to demonstrate
adequate method performance in their own laboratory (and/or in a qualified
third-party contract laboratory) using accepted protocols outlined in the Good
Manufacturing Practices for dietary supplements in the United States (21 CFR
Part 111) and/or by AOAC International, International Organization for
Standardization (ISO), the World Health Organization (WHO), and the
International Conference on Harmonization (ICH).
3. Common and Scientific Names
3.1 Common name: Grape
3.2 Other common names
English:
European grape, wine grape3
Chinese: Pu
tao (葡萄)†
French: Raisin
German:
Traube, Weintraube
Italian:
Uva
Spanish:
Uva
3.3 Accepted Latin binomial: Vitis vinifera L.3
3.4 Synonyms: Cissus vinifera (L.) Kuntze4,5
3.5 Botanical family:
Vitaceae
4. Botanical Description
Grapes,
the fruit of the grape vine, have been used as a source for food and beverages
for thousands of years, and are easily distinguished from adulterating species
when present in the whole form. The seeds of grapes, obtained as a by-product
from the juice or wine industry, are used fresh, or more commonly dried to
produce a liquid extract using a solvent (e.g., water, or mixtures of water
with ethanol, ethyl acetate, or acetone) which is filtered, and may be
subjected to further processing before it is typically spray-dried to obtain a
dry extract containing high levels of naturally occurring grape seed phenolic
compounds.
Table 1. Scientific names, family, and common names
of potential grape seed extract adulterants 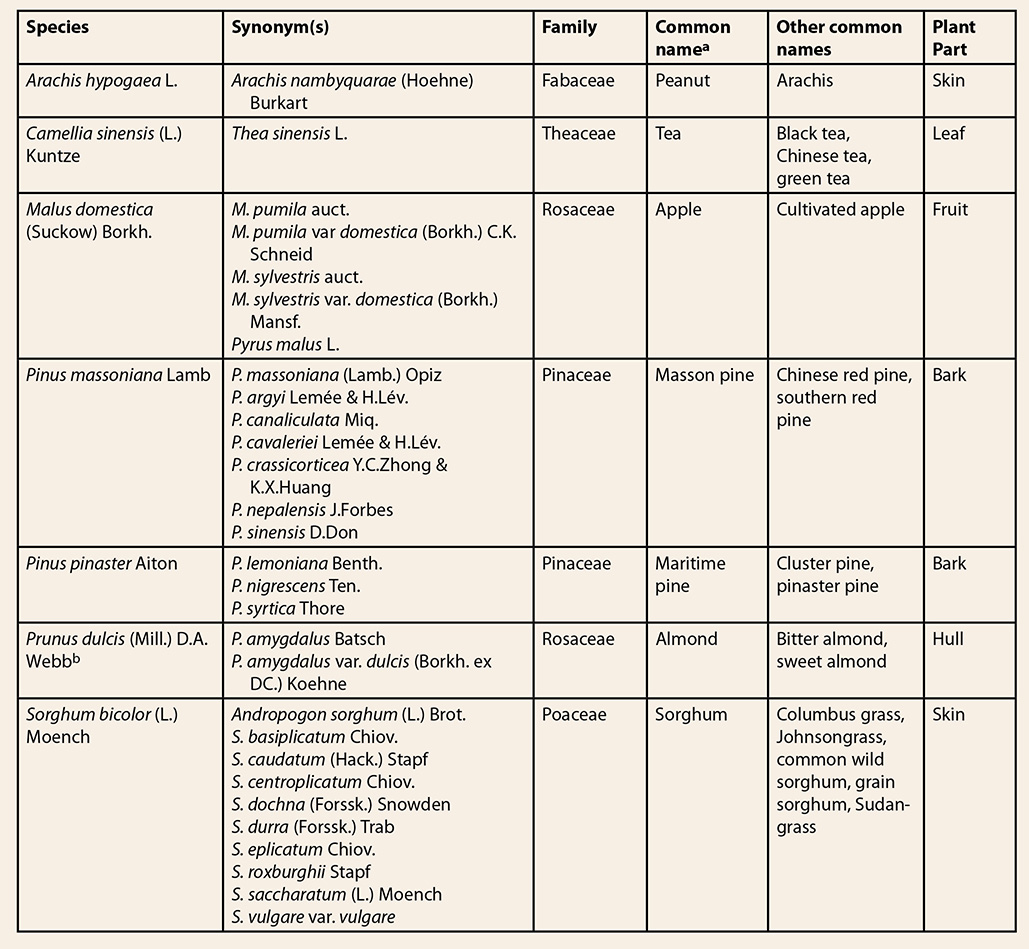
aPropelargonidin
containing extracts from non-grape seed sources.6,7 bHerbs of Commerce, 2nd
ed.3 Peanut skin extract, which is a high-volume byproduct of the
peanut industry, is less expensive and typically available at a much greater
volume than GSE. In the United States, a typical peanut mill may produce up to
17 tons of peanut skins per week, and the material was sold for as little as US
$0.02/kg in 2009.8 Costs for processed materials are still
low, e.g., in China, in 2015, the price for peanut skin extract was at
US $10-13/kg, compared to pine bark extract at US $20-22/kg, and GSE at US $30-35/kg,
although proprietary GSEs may be sold for up to US $110/kg.
Sections 5-8 of this document discuss
macroscopic, microscopic, genetic, and phytochemical authentication methods for
grape seed. A comparison among the various approaches is presented in Table 3
at the end of section 9.
5. Identification and Distinction using
Macroanatomical Characteristics
Macroscopic
identification criteria of grape seeds9,10 can be helpful to manufacturers of GSE
that purchase dried grape seeds, to avoid confusion with other dried seeds.
Macroscopic evaluation of GSEs may help to determine the absence of exogenous
anthocyanin-containing materials based on variations in the typical brown color
of GSEs. However, macroscopic identification is inadequate to authenticate GSEs
or detect adulteration.
6. Identification and Distinction using
Microanatomical Characteristics
There
are some publicly available data on the microscopic features of grape seeds.10,11 However, microscopic
distinction among GSEs and extracts of its adulterants has not been done. It is
not possible to identify these adulterants by microscopy because the extraction
process removes any characteristic cellular structures that may be used to
determine the identity of the material. Therefore, botanical microscopy is not
an appropriate means to authenticate grape seed extracts.
7. Genetic Identification and Distinction
There
are no published genetic methods to authenticate GSE, or to detect adulteration
with extracts from other plant sources. While some DNA-based methods have successfully
been able to authenticate certain dried extracts,12-15 the limitations in determining the
identity and composition of highly processed materials are well-known,14,16,17 and, as such, genetic methods are of
limited use at this time for GSE authentication or detection of adulterants.
8. Chemical Identification and
Distinction
While
there is an abundance of published analytical methods for grape juice and red
and white wines, the number of methods for authentication of GSEs, and the
detection of GSE adulteration is limited. Distinction between GSEs and their
adulterants by chemical analysis requires knowledge of the composition of the
ingredients, in particular the structures of the PACs found in grape seeds and their
adulterating species. The composition of GSEs, and extracts of their main
adulterants, is indicated below. The data are based on published literature;
however, the composition of these extracts depends on many factors, including
the geographic origin of the source material, the cultivar, and the extract
manufacturing process. Review of additional PAC types occurring in various food
and non-food ingredients has been published by Hellström et al, and Monagas et
al.18,19 The main characteristics of PACs from
grape seed and other low-cost food sources are listed in Table 2.
8.1 Chemistry of GSE and the potential adulterants
Vitis vinifera:
GSE is almost exclusively supplied to dietary supplement manufacturers in the
form of a dry extract. The extract contains phenolic compound concentrations
ranging from ca. 50 – 90% of the extract. The main phenolic compounds are
flavan-3-ol monomers and polymers and their gallic acid esters. Grape seeds
contain predominantly B-type PACs, which are flavan-3-ol polymers where the
units are linked by a single bond (Figure 1). Appeldoorn et al. isolated
procyanidin B1 ‡, B2, B3, and B4 from a
commercial GSE, accounting for 3.2%, 7.1%, 1.5%, and 1.2%, respectively, of the
extract.20 Similar results were reported by Weseler and
Bast,21 with concentrations of 7.7%, 8.3%, 2.8% and
1.6% of procyanidins B1, B2, B3, and B4, respectively. The presence of B-type
dimers, trimers, tetramers, and polymers of up to the size of a dodecamer
trigallate was described by Weber et al.22 The authors analyzed four commercial GSEs, and
found that the molecular weight distribution varied substantially depending on
the product. Average degrees of polymerization (DP) for commercial GSEs were
reportedly between 3-11,23,24 although depending on processing, the DP may deviate substantially from
these values. Other
authors have described two different types of GSE on the market, with
polyphenol contents depending on the treatment with the enzyme tannase, which
cleaves gallic acid units from molecules such as flavan-3-ols, converting e.g.,
epicatechin-3-O-gallate into
epicatechin and gallic acid. While untreated GSEs generally have gallic acid
concentrations of less than 0.5%, tannase-treated extracts have gallic acid
concentrations increased to levels between 1.75 – 4.8%, but only trace amounts
of galloylated procyanidins.7,25-28 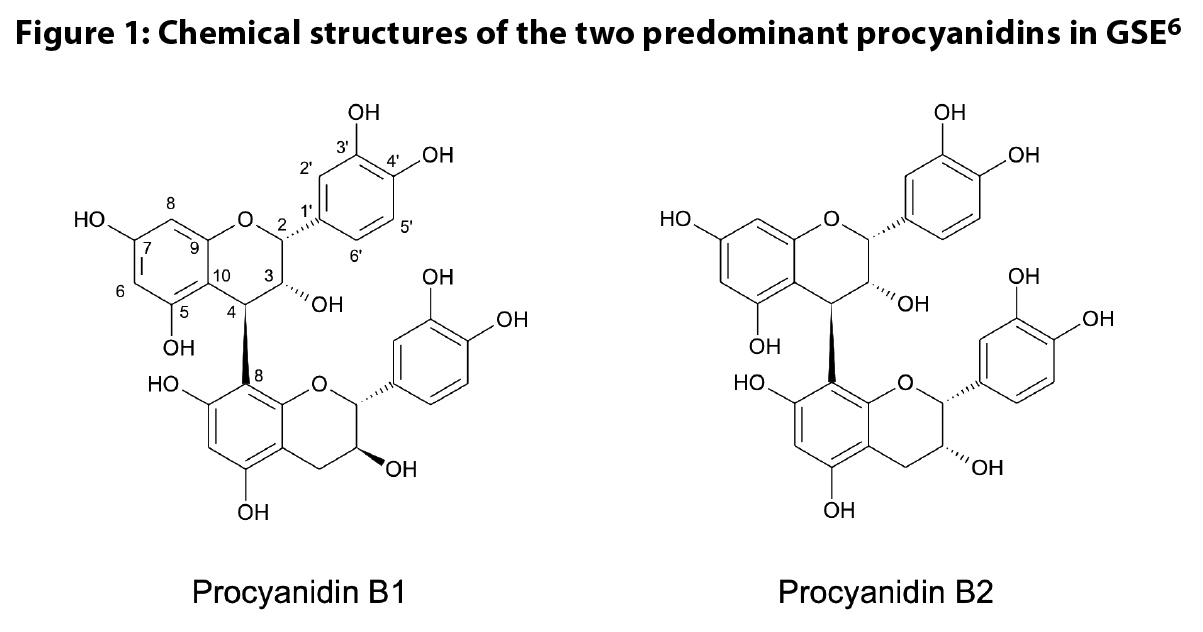 Arachis
hypogaea: Peanut skin extracts contain both A-type and B-type
PACs.29,30 Appeldoorn isolated a number of PACs
from peanut skin, with A-type dimer procyanidin A1 and A2 as most abundant
(6.9% and 2.1%, respectively).20 Procyanidin B7 was
present at 0.2%.20 Dudek et al.
confirmed the presence of procyanidins A1 and A2, and isolated four trimers and
two tetramers, named peanut procyanidins A-F. Besides procyanidin A1, peanut
procyanidin E was the most abundant in a 70% aqueous acetone extract of the
skins.31 Other phenolic compounds
in peanut skin include flavonols (quercetin, kaempferol, isorhamnetin and their
glycosides), isoflavones (genistein, hesperetin), anthocyanins (cyanidin,
cyanidin-3-O-glucoside, cyanidin-3-O-sophoroside, peonidin-3-O-galactoside, and petunidin-3-O-galactoside), and the stilbene
resveratrol.32
Camellia sinensis:
Green tea extracts are well characterized and dominated by flavan-3-ol
monomers and their gallates. The main flavan-3-ol in green tea leaves is
epigallocatechin gallate (EGCG) at 0.7-14.4%, followed by epigallocatechin (0-4.7%),
epicatechin gallate (0.2-4.2%), epicatechin (0-1.5%), catechin (0-1.2%) and
gallocatechin (0.3-0.8%).33-36 Highly purified extracts containing up
to 95% of epigallocatechin gallate (EGCG) are marketed, but concentrations in
extracts used in commercial dietary supplements are generally between 40-70%. Green
tea leaf also contains 1-3% caffeine, 0.01-0.9% theobromine, and small amounts
(< 0.1% ) of theophylline.33,34,37 In addition, the leaf contains
flavonoids, predominantly glycosides of quercetin, kaempferol, and myricetin as
3-O-glucosides, 3-O-galactosides, 3-O-rutinosides, 3-O-galactosylrutinosides,
and 3-O-glucosylrutinosides.35,38,39 The chemical profile of green tea
extract and GSE is altogether different and allows an easy distinction between
the two ingredients.
Malus domestica: The
phenolics in apple peel are composed of flavonol glycosides (e.g., hyperoside,
quercitrin, avicularin), flavan-3-ol monomers (epicatechin, catechin) and
polymers, hydroxycinnamic acids (chlorogenic acid, caffeic acid), and the
dihydrochalcone phloridzin.40,41 Red apple peels also contain
anthocyanins, mainly cyanidin-3-O-galactoside
and other cyanidin glycosides.42 The PACs have the
highest concentration of phenolics in whole apples with > 70% of all
phenolic compounds followed by the hydroxycinnamic acids (4-18%), flavonols
(1-11%), dihydrochalcones (2-6%) and anthocyanins (1-3%).42-44 Apple peels were found to contain
between 3-28.4 times more phenolic compounds than the flesh. Flavonol
glycosides are absent in the flesh, which contains mainly PACs and phenolic
acids. Epicatechin and procyanidin B2 were the main flavan-3-ols according to the
investigation by Kschonsek et al.41 The apple PACs
consist mainly of linear B-type procyanidins with epicatechin as the
predominant unit.45 According to
Feliciano et al., 88.3% of the flavan-3-ol polymers in apples are made of these
B-type PACs.46 While the
adulteration of grape seed extract with apple peel PACs does not make economic
sense, it is a good source of procyanidin B2, one of the main procyanidins in
GSE. (J. Xin [Skyherb] email communication to S. Gafner, November 25, 2018).
Pinus spp.: Weber et al.22 investigated the PAC
type and size in extracts from maritime pine and Masson pine. From an economic
perspective, Masson pine extracts are 5-10 fold less expensive than maritime
pine bark extracts, making Masson pine more attractive as an economic
adulterant (Yannick Piriou [DRT (les Dérivés Résiniques et Terpéniques)] email to Maria J. Monagas, May 3, 2018). Galloylated PACs have been reported from maritime
pine and Masson pine, but don’t seem to be abundant.22 The monomer units
consist mainly of catechin and epicatechin, although small amounts of
epigallocatechin and gallocatechin have been reported as well. 47,48 Typically, pine bark extracts contain
only B-type PACs. The average DP of a hot water extract of P. pinaster is between 6 and 7. 47,49 Similar results were reported for Scots
pine (P. sylvestris) by Bianchi et
al.50 The PAC fraction of
a hot water extract consisted of exclusively B-type procyanidins with average DP
of 6.7. A comparison of HPLC-UV fingerprints between grape seed and Masson pine
extract did not show a substantial difference, except that the Masson pine
extract had a larger concentration of more highly polymerized PACs and
exhibited the peak of an A-type dimer.7
Prunus dulcis:
The composition of almond skin extract, a byproduct of the almond industry, has
been described in a patent by Bartolome et al.51 Almond skin extract
contains PACs with afzelechin, catechin, gallocatechin, epiafzelechin, epicatechin, and
epigallocatechin as monomers. Besides catechin and epicatechin, the extract
contains mainly procyanidins B1, B2, B3, B5, B7 and C1, and unidentified A-type
dimers and trimers, although MALDI-TOF data showed presence of molecules with DPs
of up to 10. A-type dimers and trimers made up ca. 19-22% of the total amount
of PAC dimers and trimers in almond skin extract. Almond skin extract also
contains propelargonidin and prodelphinidin dimers and trimers, albeit at low
concentrations (less than 5% of the total amount of monomers, dimers, and
trimers). The main flavonoids in the skin have been reported as kaempferol-3-O-rutinside, isorhamnetin-3-O-rutinoside, isorhamnetin-3-O-glucoside, and naringenin-7-O-glucoside.52,53 The occurrence of prodelphinidins and
propelargonidins, and the absence of galloylated PACs may provide a means to
distinguish almond skin extracts from GSEs.
Sorghum bicolor: A
large number of phenolic compounds have been isolated from S. bicolor: These include hydroxybenzoic acids, hydroxycinnamic
acids, flavonoids, anthocyanins, flavan-3-ols, and flavan-4-ols.54-56 The composition of phenolic compounds
in sorghum is strongly dependent on the genotype. Ferulic and p-coumaric acids are the most abundant
phenolic acids, and are mostly bound to cell walls. High amounts of bound
protocatechuic acid were reported from white and red sorghum. The flavonoids
apigenin and luteolin are predominantly found in tan-pigmented genotypes. Other
flavonoids from sorghum include eriodictyol, eriodictyol 5-O-glucoside, taxifolin, taxifolin 7-O-glucoside, and kaempferol 3-O-rutinoside-7-O-glucuronide. Sorghum anthocyanins are
unusual by the fact that they do not contain a hydroxyl group in position 3.
The most common anthocyanins are apigeninidin and luteolinidin. Sorghums with a
black pericarp (bran) have higher amounts of anthocyanins compared to those
with red and brown pericarp. The occurrence of flavan-4-ols seems to be
restricted to red bran sorghum.54,55
Condensed
tannins are found only in sorghum genotypes II and III, which have dominant B1
and B2 genes. Sorghum PACs are mainly of the B-type with epicatechin as
extension unit and catechin as terminal unit.55,57 However, Krueger et al. reported the
presence of some variations in the PAC composition from Ruby Red sorghum (a
type III genotype), e.g., the inclusion of gallocatechin/epigallocatechin
units, PACs containing proluteolinidin and proapigenidin monomers, and the
occurrence of eriodictyol, or eriodictyol-5-O-glucoside
as terminal units.58 White sorghum
genotypes contain the monomers catechin and epicatechin, but no polymers.55,59
Table 2: Proanthocyanidin characteristics
of low-cost materials containing condensed tannins  aMeasured
by thiolysis bThe
number depends on the processing method. For grape seed, average degrees of
polymerization between 1-37 have been reported on isolated fractions.65-67
8.2 Laboratory methods
Note: Unless otherwise
noted, all methods summarized below are based only on the analysis of
the powdered GSE and its adulterants. It should also be emphasized that
there is no substitute for a strong working knowledge of PAC chemistry and
their methods of analysis when venturing into authentication work.
8.2.1 Chemical and botanical reference
materials
With
any analytical method, it is important to have reliable standards and or
reference materials. This requirement is especially compounded when conducting
investigations into adulteration. The question for the
analyst may be what potential adulterants to test for. The answers may be
logically deduced by asking what classes of compounds and their botanical
sources could be used to replace or substitute authentic GSE. Adulterants
containing gallic acid, and/or PACs would all be logical substitutes with
increasing compositional similarities to GSE. With respect to botanical
sources, waste streams from agricultural and food industries would be the most
economically attractive. In any scenario, the researcher should consider other
qualitative features of a given chromatogram noting and spectroscopically
characterizing peaks that appear inconsistent with an authentic GSE reference
material, or use the entirety of the chromatographic or
spectroscopic/spectrometric fingerprint to assess the authenticity of the
material. In addition to an authentic GSE, the analyst should compare the
results with those from extracts from rational/potential adulterating
materials. A reference grape seed extract can be obtained from the United States
Pharmacopeia (USP), while authentic grape seeds are available from
Alkemist Labs and ChromaDex. A grape seed/skin mixture is offered by the American
Herbal Pharmacopoeia (AHP). However, some of the adulterating materials may not
be easily available as botanical reference materials to quality control
personnel.
An important factor is the use of chemical reference
standards for the chromatographic assays. Afzelechin, catechin, epiafzelechin, epicatechin,
epigallocatechin, gallocatechin, and some PAC standards (e.g., procyanidins A1,
A2, B1, B2, and C1) can be sourced commercially, but many of the grape seed PAC
trimers and more highly polymerized molecules are not available from leading
manufacturers of chemical reference standards.
8.2.2 Colorimetric
assays
The
information discussed below is provided as a background for subsequent discussions
on more specific approaches to assess adulteration of GSEs. Colorimetric
methods for PACs include the Bate Smith reaction, Folin-Ciocalteu method,
butanol/hydrochloric acid (HCl) assay, vanillin assay and 4-(dimethylamino)cinnamaldehyde
(DMAC) assay. Thorough descriptions with applications, strengths, and
limitations of the various colorimetric methods are available.61,68 A summary on
colorimetric methods to measure color and anthocyanins in fruits has been
published by Wrolstad.69 This review lists
the strengths and limitations of the various methods.
Comments:
Colorimetric assays typically are simple and affordable to run. These assays utilize
reagents that react with phenolics to form colored products that are readily
quantifiable by absorption measurements. These methods are of great utility for
screening of plant materials for phenolics and to measure gross phenolic
content.
However,
colorimetric
assays are non-specific in that they measure total phenolic content (i.e., phenolic acids,
flavonoids, PACs, etc.) but fail to distinguish among molecules
that may be characteristic of a particular compound class. Since
phenolic compounds are found throughout the plant kingdom, these assays provide no
information to determine the botanical source or assess
adulteration. Regardless, these assays are commonly employed to study
GSEs,
and often represent the only chemical assay on a certificate of analysis for
ingredients that are rich in PACs. With respect to adulteration
with peanut
skin extracts, colorimetric assays have had limited use.7,70 Based on the generic nature of the approach, the utility of
colorimetry to detect the presence of adulterants in GSE is not
supported.
It should be noted that colorimetry in conjunction with protein precipitation
steps can allow for the determination of total phenolic content and those
phenolic compounds that interact with proteins. As such, a
purported GSE that does not show an interaction
with protein may be considered a low molecular fraction thereof or represent
non-authentic
material.
The analysis of total anthocyanins using the
colorimetric method by Niketic-Aleksic showed that concentrations above 0.1%
(w/w) in GSE powder can be detected.71 Therefore the simple
Total Anthocyanin colorimetric method
can easily be used as a screening tool for the presence of anthocyanins prior
to HPLC analysis. Residues from grape skin may make their way into GSEs, but
unintentional inclusion of grape skin materials is expected to be at low
amounts and therefore lead to anthocyanin concentrations below the 0.1%
mentioned above. Presence
of anthocyanins in GSE above 0.1% is an indication of
adulteration.
8.2.3
TLC/HPTLC
Methods
described in the following literature were covered in this review: Lea and Arnold,72 Villani et al.,7 and Sudberg et al.70
Comments: One of the first thin layer
chromatographic (TLC) separations of procyanidins according to their DP was carried
out by Lea and Arnold in 1978 using apple ciders.72 Villani et al.7 developed a new TLC
method in 2015 for a quick and inexpensive qualitative test to detect presence
of peanut skin and pine bark extracts in GSEs. A mixture of acetone-acetic acid-toluene
was used to develop the samples on a silica TLC plate. A-type PAC dimers were used
as markers for peanut skin. This approach can be used to easily determine the
presence of A-type PAC dimers in GSEs. However, the authors noted that given
similar chemistries, the method does not allow for pine bark and GSE
differentiation.
High-performance
thin-layer chromatography (HPTLC) was successfully used to detect adulteration
with peanut skin extract in commercial GSEs by Sudberg et al.70 Catechin, authentic
grape seed, and peanut skin extracts were used as reference materials. Separations were effected over silica gel
plates using a toluene: acetone: formic acid mobile phase. Bands were observed
under visible light post treatment with Fast Blue Salt B derivatizing reagent
(Figure 2). The validated HPTLC approach described is a viable diagnostic tool
for readily assessing the presence of PACs that typify peanut skin extracts. Limits
of visual detection of presence of peanut skin detection were approximated at
5% (w/w).
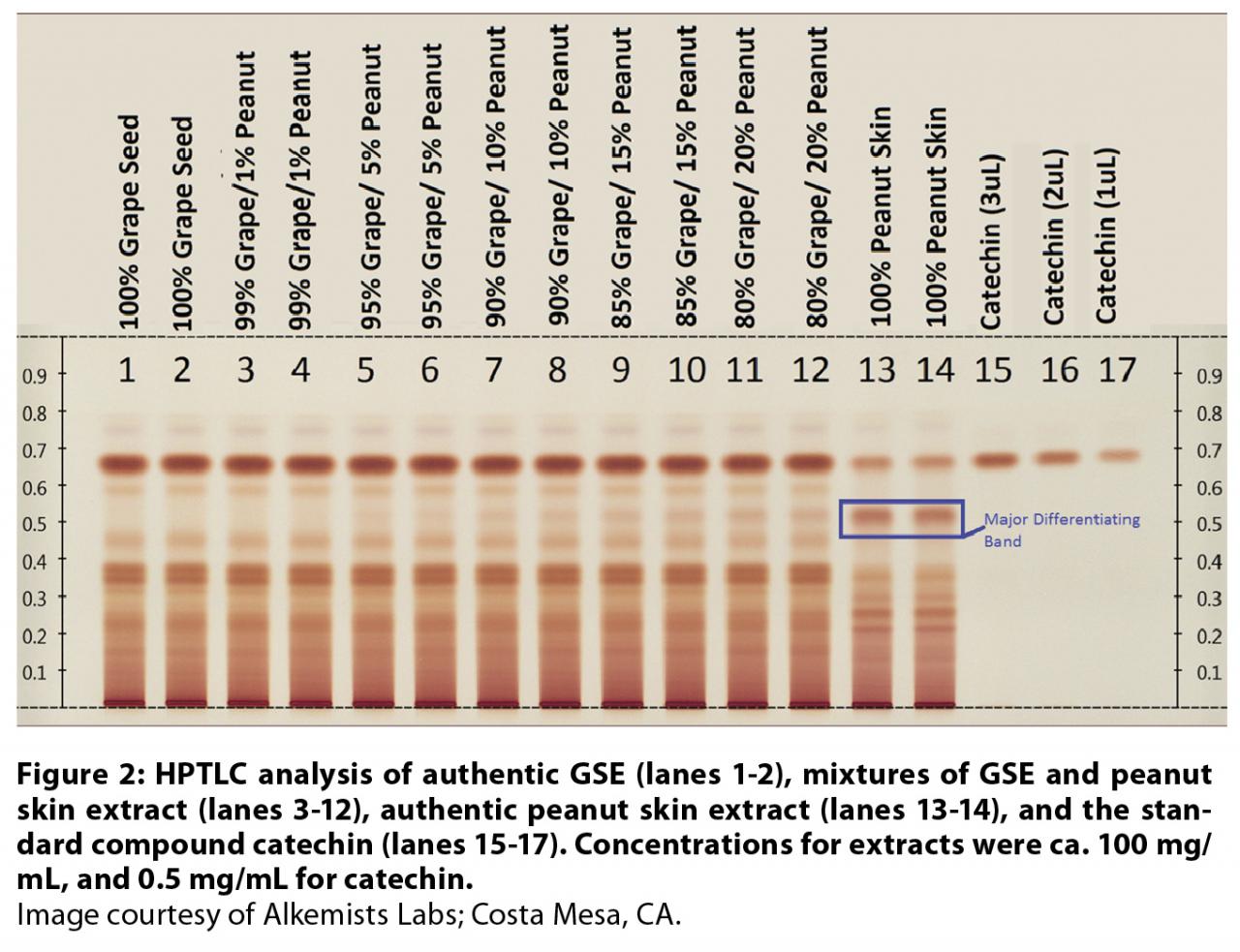
8.2.4 HPLC
Numerous
high-performance liquid chromatographic (HPLC) methods utilizing a range of
stationary phases (e.g., C18, silica, diol, cyano, amide, various polymeric phases,
etc.) and detectors (e.g., ultraviolet [UV], photodiode array [PDA], fluorescence
[FLD], mass spectrometric [MS], coulometric, etc.) were utilized for the
analyses of PACs and anthocyanins from various botanical sources. The sample preparation
for both PACs and anthocyanins is largely matrix dependent.
Regarding
PACs, some materials may require a defatting step to remove lipids and waxes
prior to extraction. Extraction solvents can range from mixtures of aqueous
alcohol or acetone or acidified versions thereof. Many commercial botanical
extracts typically require only dissolution in an HPLC-compatible solvent and
filtration prior to injection. Solid phase extraction may be required to remove
interfering or other extraneous materials. There are essentially two approaches
to analysis; destructive and non-destructive. The former approach can involve
acid catalyzed cleavage of a sample in the presence of nucleophiles (e.g.,
benzyl mercaptan, phloroglucinol, etc.). The HPLC analysis of reaction products
provides qualitative information with respect to subunit
structure (e.g., flavan-3-ol
substitution pattern) and therefore allows the user to determine the presence
of atypical PACs (e.g., propelargonidins) in a suspect GSE. Thiolysis is one
widely used approach that is undertaken in the characterization of PACs,73 and to calculate the
average DP. Non-destructive methods analyze the GSEs without prior chemical
reaction. The disadvantage of non-destructive HPLC methods is the often poor
resolution of PAC molecules with four or more units, in particular in methods
using reverse phase chromatography, giving yield to broad humps of unseparated
PAC-polymers that are useless for species distinction. The use of
polyvinylpolypyrrolidone to bind polyphenols, or filters with a molecular
weight of 3000 or 5000 Da to remove the larger PACs prior to analysis may
improve the chromatograms to some extent,28,74 but these sample preparation steps
carry the risk of eliminating potentially characteristic molecules as well. Both
approaches rely on analytical standards or reference materials for qualitative
and quantitative HPLC analyses. The combination of both approaches is
complementary in evaluating GSEs for adulterants.
A crucial part of an HPLC identity test involves
comparative HPLC of intact suspect and authentic samples (see 8.2.1 and Figure
3). As stated above, the analyst may use an array of separation modes. One of the
earliest examples of HPLC use is the normal phase separation of grape seed
procyanidins by DP using a gradient of acidified methanol in dichloromethane. 75 Later, Waterhouse et al. 76 applied the same conditions using a cacao
extract as a reference material to approximate the DP in GSEs. 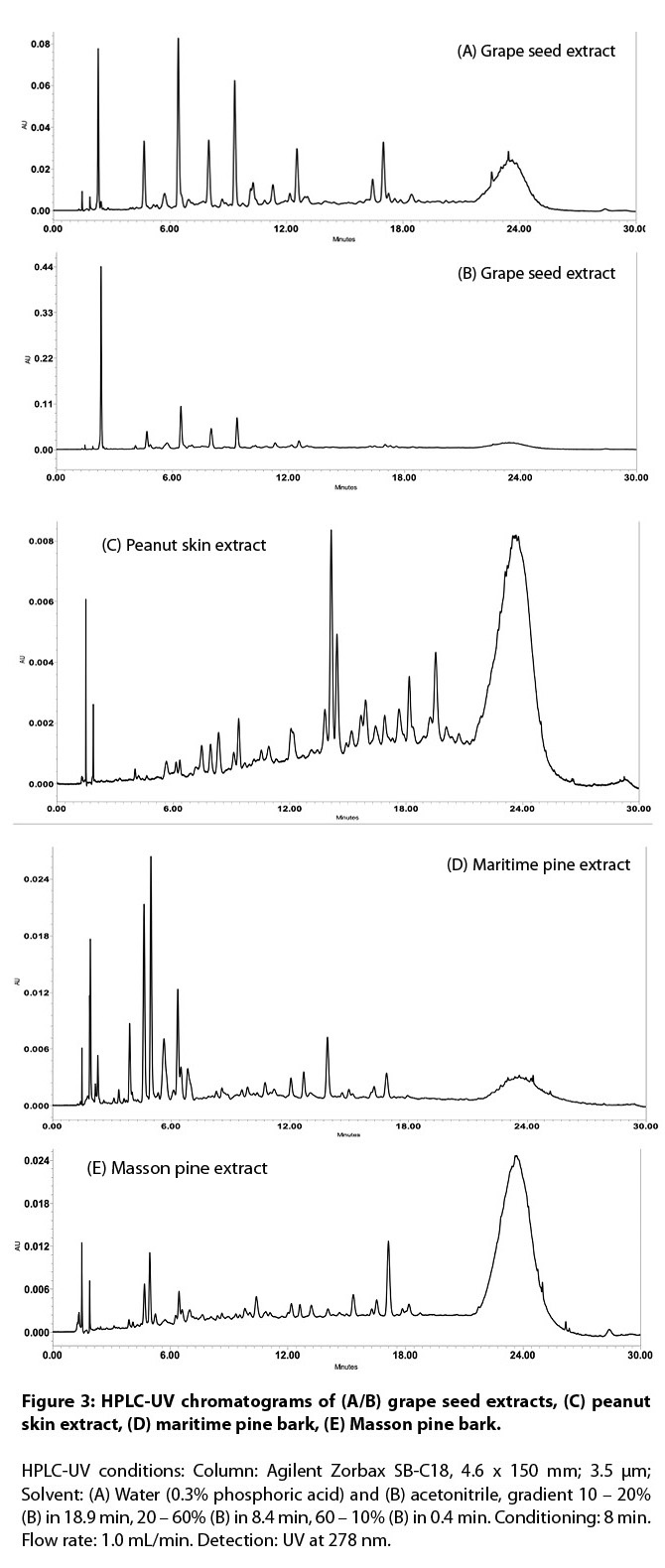
To obtain
separation of PAC molecules according to size, gel permeation chromatography
(GPC) may also be employed. However, this approach tends to suffer from poor
resolution and a need for derivatization. Elimination of derivatization and
minor improvements in resolution were achieved by Kennedy and Taylor whereby
fractionated GSEs were separated over two porous polystyrene–divinylbenzene
columns connected in series.77
With
the goal of eliminating the use of methylene chloride and minimizing
florescence quenching as was the case with Rigaud’s silica method,75 a hydrophilic
interaction chromatographic (HILIC) method was developed by Kelm et al.78 to separate cacao
procyanidins based on DP. Separation was achieved by an acidified gradient of
methanol into acetonitrile over a diol stationary phase. Normal phase and HILIC
separations formed the basis of methods aimed at MS characterization of GSEs
described below.
8.2.5
HPLC-Vis
The methods described by Wang et al.,79 and Oh et al.80 were evaluated and
used in this review.
Comments: The publications
describe the separation and identification of anthocyanins using HPLC-Vis using
a photo diode array detector and HPLC-MS. Anthocyanins are generally detected
at wavelengths between 520-546 nm.81 These pigments are
not found naturally in grape seeds, but trace amounts may be transferred on the
grape seed from the process of crushing and separation from red grape skins
(see section 8.2.2). The brownish colored GSE would be visually reddish purple in
color if large amounts of anthocyanins were transferred into the GSE extract.
8.2.6
HPLC-UV/FLD
Methods described in the following literature
were evaluated in this review: Kelm et al.,78 Nakamura et al.,25 and Robbins et al.82
Comments: While
the addition of a FLD reportedly leads to a better signal-to-noise ratio for
catechin monomers, dimers, and trimers compared to HPLC-UV,25 data on the ability
of HPLC-FLD to distinguish grape seed extracts from its adulterants are
lacking.
8.2.7
HPLC-MS, UHPLC-MS, HPLC-UV/MS, UHPLC-UV/MS, and HPLC-MS/MS
Comments: Methods described in the
following literature were discussed in this review: Appeldoorn et al.,20 Gu et al.,73 Kelm et al.,83 Kuhnert et al.,84 Li et al.,85 Ma et al.,86 Sica et al.,28 Villani et al.,7 and Zhang et al.87
The
combination of normal or hydrophilic interaction (HILIC) phase separations by
DP coupled with MS offers a powerful qualitative tool to characterize and
assess the distribution of monomers, oligomeric sets and polymers. Ambiguity of
closely-eluting trimers and their galloylated forms are largely negated when
one can differentiate between masses. These and other separation modes (i.e.,
amide-C18) can also be used to elucidate the presence of adulterants.
Kelm
et al. extended their earlier work to the separation of grape seed tannins over
a diol stationary phase: ESI-MS analysis confirmed the presence of procyanidin
dimers through octamers with 0 to 3 units of galloylation.83 Kuhnert et al. essentially
adapted this approach for separation and characterization of dimers through
pentamers. Characterization and semi-quantification was achieved by aid of a
tetramer standard.84 However, no attempt
was made to evaluate these conditions for the ability to detect adulteration.
Appeldoorn
utilized normal and reverse phase HPLC-MS to characterize various A- and B-type
PACs derived from grape seed and peanut skins. Since the conditions were
optimized for isolation of PACs, it is not clear how well these conditions are
suited for authentication purposes.20
Villani et al., used high-performance liquid
chromatography with ultraviolet and mass spectrometric detection (HPLC-UV/MS)
to obtain a chemical fingerprint of grape seed, peanut skin, and pine extracts.7 Due to the
similarity of GSE and pine (P. massoniana)
bark extract, distinction between the two materials is difficult, in particular
in case of grape seed and pine bark extract mixtures. Peanut skin is readily
distinguished from GSE using HPLC-UV/MS.
Diol
HPLC and HPLC-MS/MS analyses were used by Kelm et al. to characterize 20 authentic
and commercially obtained bulk materials labeled as GSEs.6 Atypical peaks
observed in HPLC profiles were further evaluated by HPLC-MS/MS, which lead to
the detection of both A-type procyanidins and B-type propelargonidins in one
and four samples, respectively. Therefore, this approach can be used to detect
adulteration with extracts containing A-type procyanidins and/or
propelargonidins.
Li
et al., (2002) utilized HPLC-atmospheric-pressure chemical ionization (APCI)-MS
to characterize gallic acid, dimers procyanidin B4 and B2, (+)-catechin, (-)-epicatechin,
and (+)-epicatechin gallate in grape seed extract.85 Despite the lack of
any structural information of DP>2, the presence of procyanidins B2 and B4
dimers could serve as ancillary information in the authentication of GSE.
In
2018, Ma et al., identified epicatechin vanillate in grape seed and red wine at
ppb levels using HPLC-HRMS/MS.86 Given the
peculiarity of this compound, it may serve as a unique marker compound for
grape seed extracts. However, a more thorough survey of authentic grape seed
extracts would need to be undertaken in order to affirm its universal presence
in grape seeds, and to evaluate the usefulness of the method in GSE
authentication.
Zhang
et al.87 developed a 65 min.
long ultra high-performance liquid chromatography combined with a high
resolution mass spectrometric detection (UHPLC-HRMS) method using a computer
algorithm to extract, identify and quantify catechin monomers and oligomers up
to octamers. The approach allowed distinguishing grape seed PACs from those of
apple, chocolate, and other fruit samples. Based on the available data, HPLC-MS
methods can distinguish among grape seed extracts and its adulterants. Ideally,
these methods are combined with a robust chemometric analysis. More samples
need to be included to account for the variability in extracts due to natural
variations and differences in the manufacturing processes.
The paper by Sica et al. describes a rather
comprehensive characterization of GSE using ultrahigh-performance liquid
chromatography–ultraviolet–charged aerosol detector–high resolution mass
spectrometry (UHPLC-UV-CAD-HRMS). A rough separation of the GSE constituents
was achieved on a C18 column in 75 min. Using the CAD chromatogram, GSE was
easily distinguished from peanut skin and maritime pine by comparison of the
chromatographic fingerprint. The 39 major peaks of the chromatogram were
partially or completely assigned to 83 different compounds, including a broad
hump comprising the PACs with a DP of 6 or higher that was considered as one
single compound. Structural assignment was based on comparing high-resolution
MS data to those of known compounds or literature values, and by using MS fragmentation
patterns of known compounds to assign molecules to a specific class of
compounds. The authors noted an important difference between untreated and
enzymatically-treated GSEs, but were able to distinguish both types of GSEs
from the potential adulterants.
Comments: The HPLC-MS methods evaluated were mainly
developed to identify the various PACs in GSE and other materials. As such, it
is not clear how well these methods would work as routine quality control
assays to authenticate GSE, and to detect potential adulterants. The methods
published by Kelm et al., 6 Zhang et al., 87 Sica et al., 28 and Villani et al. 7 indicate that comparison of the GSE
chromatographic fingerprint with those of adulterating materials will allow
detection with peanut skin (and other ingredients having A-type PACs) and
propelargonidin-containing materials. The use of HILIC chromatography to
separate PACs is promising, but more data are needed to evaluate its ability to
confirm the authenticity of GSE.
8.2.8
MALDI-TOF
Methods described in the following literature
were evaluated in this review: Ricci et al.,88 Weber et al.,22 Hümmer and Schreier,89 and Yang and Chien.90
Comments: Matrix-assisted
laser desorption/ionization-time-of-flight (MALDI-TOF) mass spectrometry is
commonly used in research settings to determine the general structural features
(e.g., type(s) of monomer [although stereochemical assignments cannot be made
using MALDI-TOF], A-type or B-type PAC, number of monomer units, and extent of
galloylation) and distribution of PACs of various DPs within materials
containing condensed tannins. The sample preparation is quick and easy, and
consists mainly of dissolving the extract in methanol or an acetone-water
mixture and then diluting it 1:1 in the matrix system (most often a methanolic
solution of 2,5-dihydrobenzoic acid). Both, linear and reflectron modes can be
used; a comparison between the two suggests that linear mode provides better
data on PACs with a high DP.22 MALDI-TOF has shown
to distinguish among GSE, oak (Quercus
robur, Fagaceae) bark, and green tea extract,88 as well as between GSE
and pine bark extract.22 The main
disadvantage is the cost of the instrument ($180,000 – 350,000) and a lack of MALDI-TOF
capacity in many contract labs.
8.2.9
NMR
The following method was evaluated in this
review: Anstasiadi et al.91
1N and/or 13C Nuclear Magnetic Resonance (NMR) spectroscopy may be used
for comparison of suspect samples against authentic samples. Obvious
discrepancies inconsistent with proanthocyanidin molecules such as missing carbonyl
carbon peaks or methine protons at the 2 and 3 carbons on the flavanol C-ring
would suggest admixture or substitution with extraneous, non PAC-containing
materials. When coupled to pattern recognition software, NMR is a powerful and rugged
tool for the characterization and authentication of botanical extracts. With
regards to grape procyanidins, NMR has been used to effectively fingerprint and
differentiate via principle component analysis, wines by variety, vintage and
region. 91 Accordingly, it would stand to reason that a
combination of chemometrics and NMR spectroscopy could be used to detect
presence of adulterants in a GSE. However, the ability of NMR to discriminate
between GSE and PAC-containing materials still needs to be demonstrated using a
validated method.
9. Conclusions
Authentication
of GSE can be challenging. Authentication or detection of adulteration in
extracts may require more than one method. Adulteration with PACs represents a
level of sophistication discernable only by chromatographic approaches
hyphenated with a variety of detection method (UV-Vis, fluorescence detector
[FLD], mass spectrometry [MS], tandem mass spectrometry [MS/MS], etc.), or by
stand-alone spectroscopic/spectrometric methods such as nuclear magnetic
resonance (NMR) or matrix-assisted laser desorption/ionization time-of-flight
[MALDI-TOF] MS. However, adulteration with B type procyanidin-rich materials
presents a greater challenge not readily resolved by chromatographic or
spectroscopic/spectrometric methods. The best results are obtained when
chemical fingerprints, e.g., those obtained by HPLC-UV, HPLC-MS, or MALDI-TOF,
are compared against authentic GSE, as well as the potential adulterating
materials, and analyzed using state-of-the-art statistical software. Table 3. Comparison among the different approaches to authenticate grape seed extract 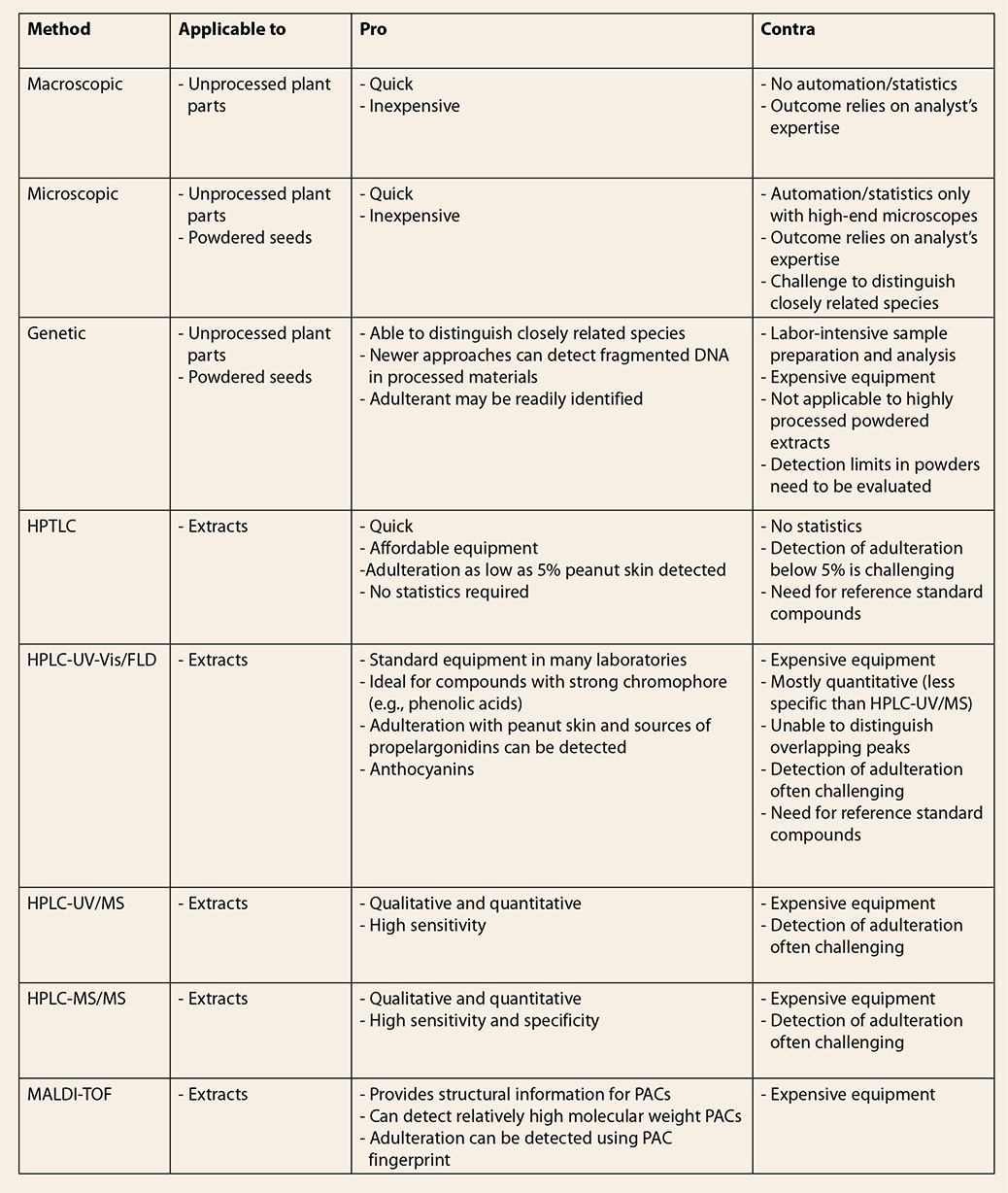
* The acronym GSE should not be confused with the acronym GFSE, referring to Grapefruit Seed Extract, which is an entirely different material. In some original publicaitons on GFSE adulteration, the authors use "GSE" to refer to grapefruit seed extract. † Grape seed in Chinese is known as pu tao zi (葡萄籽). ‡The terms proanthocyanidin and
procyanidin seem to be used interchangeably in the literature. However, proanthocyanidin is a generic term for a family of
structurally related polyphenolic compounds comprised of the procyanidins,
prodelphinidins, propelargonidins, etc. The different proanthocyanidin classes
are distinguished by the specific flavan-3-ol hydroxylation pattern, e.g.,
3,3’,4’,5,7-pentahydroxyflavan-3-ol in case of the procyanidins, or
3,4’,5,7-tetrahydroxyflavan-3-ol for the propelargonidins. The name
“proanthocyanidin” is derived from the fact that these compounds produce
anthocyanidins when treated with a mineral acid. Specifically, a procyanidin
will produce the anthocyanidin cyanidin, a prodelphinidin will yield the
anthocyanidin delphinidin, a propelargonidin will be converted into
pelargonidin, etc.
10. References
- Feringa
HHH, Laskey DA, Dickson JE, Coleman CI. The effect of grape seed extract on
cardiovascular risk markers: A meta-analysis of randomized controlled trials. J Am Diet Assoc. 2011;111(8):1173-1181.
- Ma Z, Zhang H. Phytochemical constituents, health
benefits, and industrial applications of grape seeds: A mini-review. Antioxidants. 2017;6(3):71.
- McGuffin M, Kartesz JT, Leung AY, Tucker AO. Herbs of Commerce. 2nd ed. Silver Springs,
MD: American Herbal Products Association; 2000.
- Tropicos.org. Missouri Botanical Garden. https://www.tropicos.org/Name/34000217.
Accessed January 17, 2019.
- The Plant List (2013). Version 1.1. http://www.theplantlist.org/tpl1.1/record/kew-2457006.
Accessed January 17, 2019.
- Kelm MA, Kupina S, Shrikhande A. Grape seed
extract authentication. 250th American Chemical Society National Meeting &
Exposition; 2015; Boston, MA.
- Villani TS, Reichert W, Ferruzzi MG, Pasinetti GM,
Simon JE, Wu Q. Chemical investigation of commercial grape seed derived products
to assess quality and detect adulteration. Food
Chem. 2015;170:271-280.
- Reed KA. Identification
of phenolic compounds from peanut skin using HPLC-MSn. Blacksburg, VA,
Virginia Polytechnic Institute and State University; 2009.
- Cadot Y, Miñana-Castelló MT, Chevalier M.
Anatomical, histological, and histochemical changes in grape seeds from Vitis vinifera L. cv Cabernet franc
during fruit development. J Agric Food
Chem. 2006;54(24):9206-9215.
- Nowshehri JA, Bhat ZA, Shah MY. Pharmacognostic
standardisation and phytochemical evaluation on the seeds of two Vitis vinefera L. varieties grown in
Kashmir valley, India. Pharmacogn J. 2016;8(5):465-470.
- Vitis
vinifera (seed). American Herbal Products Association; 2014. http://www.botanicalauthentication.org/index.php.
Accessed January 17, 2019.
- Wang X, Liu Y, Wang L, Han J, Chen S. A nucleotide
signature for the identification of Angelicae
sinensis radix (danggui) and its products. Sci Rep. 2016;6:34940.
- Little DP. Authentication of Ginkgo biloba herbal dietary supplements using DNA barcoding. Genome. 2014;57(9):513-516.
- Ivanova NV, Kuzmina ML, Braukmann TWA, Borisenko
AV, Zakharov EV. Authentication of herbal supplements using next-generation
sequencing. PLoS ONE. 2016;11(5):e0156426.
.
- Lu Z, Rubinsky M, Babajanian S, Zhang Y, Chang P,
Swanson G. Visualization of DNA in highly processed botanical materials. Food Chem. 2018;245:1042-1051.
- Reynaud DT, Mishler BD, Neal-Kababick J, Brown PN.
The capabilities and limitations of DNA barcoding of botanical dietary
supplements [white paper]. 2015; https://gallery.mailchimp.com/2d47ec72fa1542de734a46f71/files/Reynaud_DNA_Barcoding_White_Paper.pdf.
Accessed June 28, 2018.
- Parveen I, Gafner S, Techen N, Murch SJ, Khan IA.
DNA Barcoding for the identification of botanicals in herbal medicine and dietary
supplements: strengths and limitations. Planta
Med. 2016;82(14):1225-1235.
- Monagas M, Quintanilla-López JE, Gómez-Cordovés C,
Bartolomé B, Lebrón-Aguilar R. MALDI-TOF MS analysis of plant
proanthocyanidins. J Pharm Biomed Anal. 2010;51(2):358-372.
- Hellström JK, Törrönen AR, Mattila PH.
Proanthocyanidins in common food products of plant origin. J Agric Food Chem. 2009;57(17):7899-7906.
- Appeldoorn MM, Sanders M, Vincken J-P, et al.
Efficient isolation of major procyanidin A-type dimers from peanut skins and B-type
dimers from grape seeds. Food Chem. 2009;117(4):713-720.
- Weseler AR, Bast A. Masquelier’s grape seed
extract: from basic flavonoid research to a well-characterized food supplement
with health benefits. Nutr J 2017;16(1):5.
- Weber HA, Hodges AE, Guthrie JR, et al. Comparison
of proanthocyanidins in commercial antioxidants: grape seed and pine bark
extracts. J Agric Food Chem. 2007;55(1):148-156.
- La VD, Bergeron C, Gafner S, Grenier D. Grape seed
extract suppresses lipopolysaccharide‐induced matrix metalloproteinase
(MMP) secretion by macrophages and inhibits human MMP-1 and -9
activities. J Periodontol. 2009;80(11):1875-1882.
- Monagas M, Hernández-Ledesma B, Garrido I, J
Martín-Alvarez P, Gómez-Cordovés C, Bartolomé B. Quality assessment of
commercial dietary antioxidant products from Vitis vinifera L. grape seeds. Nutr
Cancer. 2005;53(2):244-254.
- Nakamura Y, Tsuji S, Tonogai Y. Analysis of
proanthocyanidins in grape seed extracts, health foods and grape seed oils. J Health Sci. 2003;49:45-54.
- Chamorro S, Viveros A, Alvarez I, Vega E, Brenes
A. Changes in polyphenol and polysaccharide content of grape seed extract and
grape pomace after enzymatic treatment. Food
Chem. 2012;133(2):308-314.
- Ras RT, Zock PI, Zebregs YEMP, Johnston NR, Webb
DJ, Draijer R. Effect of polyphenol-rich grape seed extract on ambulatory blood
pressure in subjects with pre- and stage I hypertension. Br J Nutr. 2013;110:2234-2241.
- Sica VP, Mahony C, Baker TR. Multi-detector
characterization of grape seed extract to enable in silico safety assessment. Front Chem. 2018;6(334).
- O’Keefe SF, Wang H. Effects of peanut skin extract
on quality and storage stability of beef products. Meat Sci. 2006;73(2):278-286.
- Constanza KE, White BL, Davis JP, Sanders TH, Dean
LL. Value-added processing of peanut skins: antioxidant capacity, total
phenolics, and procyanidin content of spray-dried extracts. J Agric Food Chem. 2012;60(43):10776-10783.
- Dudek MK, Gliński VB, Davey MH, Sliva D, Kaźmierski
S, Gliński JA. Trimeric and tetrameric A-type procyanidins from peanut skins. J Nat Prod. 2017;80(2):415-426.
- Bansode RR, Randolph P, Ahmedna M, et al.
Bioavailability of polyphenols from peanut skin extract associated with plasma
lipid lowering function. Food Chem. 2014;148:24-29.
- Lee L-S, Kim S-H, Kim Y-B, Kim Y-C. Quantitative
analysis of major constituents in green tea with different plucking periods and
their antioxidant activity. Molecules. 2014;19(7):9173.
- Friedman M, Levin CE, Choi SH, Kozukue E, Kozukue N. HPLC
analysis of catechins, theaflavins, and alkaloids in commercial teas and green
tea dietary supplements: comparison of water and 80% ethanol/water extracts. J Food Sci. 2006;71(6):C328-C337.
- Zhao Y, Chen P, Lin L, Harnly JM, Yu L, Li Z.
Tentative identification, quantitation, and principal component analysis of
green pu-erh, green, and white teas using UPLC/DAD/MS. Food Chem. 2011;126(3):1269-1277.
- Roman MC, Hildreth J, Bannister S. Determination
of catechins and caffeine in Camillia
sinensis raw materials, extracts, and dietary supplements by HPLC-UV:
Single-laboratory validation. J AOAC Int.
2013;96(5):933-941.
- Blaschek W, Frohne D, Loew D. Theae viridis folium. In: Blaschek W, ed. Wichtl – Teedrogen und Phytopharmaka. Stuttgart, Germany:
Wissenschaftliche Verlagsgesellschaft mbH; 2016:644-646.
- Lin L-Z, Chen P, Harnly JM. New phenolic
components and chromatographic profiles of green and fermented teas. J Agric Food Chem. 2008;56(17):8130-8140.
- Del Rio D, Stewart AJ, Mullen W, et al. HPLC-MSn
analysis of phenolic compounds and purine alkaloids in green and black tea. J Agric Food Chem. 2004;52(10):2807-2815.
- Pastene E, Troncoso M, Figueroa G, Alarcón J,
Speisky H. Association between polymerization degree of apple peel polyphenols
and inhibition of Helicobacter pylori urease.
J Agric Food Chem. 2009;57(2):416-424.
- Kschonsek J, Wolfram T, Stöckl A, Böhm V.
Polyphenolic compounds analysis of old and new apple cultivars and contribution
of polyphenolic profile to the in vitro antioxidant capacity. Antioxidants. 2018;7(1):20.
- Vrhovsek U, Rigo A, Tonon D, Mattivi F.
Quantitation of polyphenols in different apple varieties. J Agric Food Chem. 2004;52(21):6532-6538.
- Jakobek L, García-Villalba R, Tomás-Barberán FA.
Polyphenolic characterisation of old local apple varieties from Southeastern
European region. J Food Comp Anal. 2013;31(2):199-211.
- Guyot S, Marnet N, Laraba D, Sanoner P, Drilleau
J-F. Reversed-phase HPLC following thiolysis for quantitative estimation and
characterization of the four main classes of phenolic compounds in different
tissue zones of a French cider apple variety (Malus domestica var. Kermerrien). J Agric Food Chem. 1998;46(5):1698-1705.
- Shoji T, Mutsuga M, Nakamura T, Kanda T, Akiyama
H, Goda Y. Isolation and structural elucidation of some procyanidins from apple
by low-temperature nuclear magnetic resonance. J Agric Food Chem. 2003;51(13):3806-3813.
- Feliciano RP, Meudt JJ, Shanmuganayagam D, Krueger
CG, Reed JD. Ratio of “A-type” to “B-type” proanthocyanidin interflavan bonds
affects extra-intestinal pathogenic Escherichia
coli invasion of gut epithelial cells. J
Agric Food Chem. 2014;62(18):3919-3925.
- Kim SM, Kang S-W, Jeon J-S, Um B-H. A comparison
of Pycnogenol® and bark extracts from Pinus thunbergii and Pinus
densiflora: extractability, antioxidant activity and proanthocyanidin
composition. J Med Plants Res. 2012;6(14):2839-2849.
- Navarrete P, Pizzi A, Pasch H, Rode K, Delmotte L.
MALDI-TOF and 13C NMR characterization of maritime pine industrial tannin
extract. Ind Crops Prod. 2010;32(2):105-110.
- Jerez M, Pinelo M, Sineiro J, Núñez MJ. Influence
of extraction conditions on phenolic yields from pine bark: assessment of
procyanidins polymerization degree by thiolysis. Food Chem. 2006;94(3):406-414.
- Bianchi S, Kroslakova I, Janzon R, Mayer I, Saake
B, Pichelin F. Characterization of condensed tannins and carbohydrates in hot
water bark extracts of European softwood species. Phytochemistry. 2015;120:53-61.
- Bartolome SB, Monagas JM, Lafuente IG, et al.,
Inventors; Consejo Superior De Investigaciones Cientificas, Madrid (Spain);
Exxentia Grupo Fitoterapeutico S.A., Madrid (Spain) assignee. Phenolic extracts
of almond peel containing procyanidins, propelargonidins, and prodelphinidins,
and method for preparation thereof. 2010.
- Monagas M, Garrido I, Lebrón-Aguilar R, Bartolome
B, Gómez-Cordovés C. Almond (Prunus
dulcis (Mill.) D.A. Webb) skins as a potential source of bioactive
polyphenols. J Agric Food Chem. 2007;55(21):8498-8507.
- Bolling BW. Almond polyphenols: methods of
analysis, contribution to food quality, and health promotion. Compr Rev Food Sci Food Saf. 2017;16(3):346-368.
- Dykes L, Rooney LW. Phenolic compounds in cereal
grains and their health benefits. Cereal
Foods World. 2007;52(3):105-111.
- Dykes L, Rooney LW. Sorghum and millet phenols and
antioxidants. J Cereal Sci. 2006;44(3):236-251.
- Yang L, Allred CD, Awika JM. Emerging evidence on
the role of estrogenic sorghum flavonoids in colon cancer prevention. Cereal Foods World. 2014;59(5):244-251.
- Gu L, Kelm MA, Hammerstone JF, et al.
Concentrations of proanthocyanidins in common foods and estimations of normal
consumption. J Nutr. 2004;134(3):613-617.
- Krueger CG, Vestling MM, Reed JD. Matrix-assisted
laser desorption/ionization time-of-flight mass spectrometry of
heteropolyflavan-3-ols and glucosylated heteropolyflavans in sorghum [Sorghum bicolor (L.) Moench]. J Agric Food Chem. 2003;51(3):538-543.
- Bröhan M, Jerkovic V, Wilmotte R, Collin S.
Catechins and derived procyanidins in red and white sorghum: their
contributions to antioxidant activity. J
Inst Brew. 2011;117(4):600-607.
- Labarbe B, Cheynier V, Brossaud F, Souquet J-M,
Moutounet M. Quantitative fractionation of grape proanthocyanidins according to
their degree of polymerization. J Agric
Food Chem. 1999;47(7):2719-2723.
- Prior RL, Gu L. Occurrence and biological
significance of proanthocyanidins in the American diet. Phytochemistry. 2005;66(18):2264-2280.
- Oszmiański J, Wolniak M, Wojdyło A, Wawer I.
Influence of apple pureé preparation and storage on polyphenol contents and
antioxidant activity. Food Chem. 2008;107(4):1473-1484.
- Jiang X, Liu Y, Wu Y, et al. Analysis of
accumulation patterns and preliminary study on the condensation mechanism of
proanthocyanidins in the tea plant [Camellia
sinensis]. Sci Rep. 2015;5:8742.
- Sarnoski PJ, Johnson JV, Reed KA, Tanko JM,
O’Keefe SF. Separation and characterisation of proanthocyanidins in Virginia
type peanut skins by LC–MSn. Food Chem. 2012;131(3):927-939.
- Prieur C, Rigaud J, Cheynier V, Moutounet M.
Oligomeric and polymeric procyanidins from grape seeds. Phytochemistry. 1994;36(3):781-784.
- Sun B, Leandro C, Ricardo da Silva JM, Spranger I.
Separation of grape and wine proanthocyanidins according to their degree of
polymerization. J Agric Food Chem. 1998;46(4):1390-1396.
- Spranger I, Sun B, Mateus AM, de Freitas V,
Ricardo da Silva JM. Chemical characterization and antioxidant activities of
oligomeric and polymeric procyanidin fractions from grape seeds. Food Chem. 2008;108(2):519-532.
- Hagerman AE. The
Tannin Handbook. 2011; http://www.users.miamioh.edu/hagermae/.
Accessed June 28, 2018.
- Wrolstad RE. Color and pigment analyses in fruit
products. Vol Station Bulletin 624. Corvallis, OR: Agricultural Experiment
Station, Oregon State University; 1993:1-17.
- 7Sudberg E, Sudberg S, Nguyen J. Validation of a
high performance thin-layer chromatographic fingerprint method for the
simultaneous identification of grape seed and peanut skin and the adulteration
of commercial grape seed extract with peanut skin. AHPA (American Herbal
Products Association) Botanical Congress; 2014; Las Vegas, NV.
- Niketic-Aleksic G, Hrazdina G. Quantitative
analysis of the anthocyanin content in grape juices and wines. Lebensm Wiss Technol. 1972;5:163-165.
- Lea AGH, Arnold GM. The phenolics of ciders:
Bitterness and astringency. J Sci Food Agric.
1978;29(5):478-483.
- Gu L, Kelm MA, Hammerstone JF, et al. Screening of
foods containing proanthocyanidins and their structural characterization using
LC-MS/MS and thiolytic degradation. J
Agric Food Chem. 2003;51(25):7513-7521.
- Peng Z, Hayasaka Y, Iland PG, Sefton M, Høj P,
Waters EJ. Quantitative analysis of polymeric procyanidins (tannins) from grape
(Vitis vinifera) seeds by reverse
phase high-performance liquid chromatography. J Agric Food Chem. 2001;49(1):26-31.
- Rigaud J, Escribano-Bailon MT, Prieur C, Souquet
JM, Cheynier V. Normal-phase high-performance liquid chromatographic separation
of procyanidins from cacao beans and grape seeds. J Chromatogr A. 1993;654(2):255-260.
- Waterhouse AL, Ignelzi S, Shirley JR. A comparison
of methods for quantifying oligomeric proanthocyanidins from grape seed
extracts. Am J Enol Vitic. 2000;51(4):383-389.
- Kennedy JA, Taylor AW. Analysis of
proanthocyanidins by high-performance gel permeation chromatography. J Chromatogr A. 2003;995(1):99-107.
- Kelm MA, Johnson JC, Robbins RJ, Hammerstone JF,
Schmitz HH. High-performance liquid chromatography separation and purification
of cacao (Theobroma cacao L.)
procyanidins according to degree of polymerization using a diol stationary
phase. J Agric Food Chem. 2006;54(5):1571-1576.
- Wang H, Race EJ, Shrikhande AJ. Characterization
of anthocyanins in grape juices by ion trap liquid chromatography-mass
spectrometry. J Agric Food Chem. 2003;51(7):1839-1844.
- Oh YS, Lee JH, Yoon SH, et al. Characterization
and quantification of anthocyanins in grape juices obtained from the grapes
cultivated in Korea by HPLC/DAD, HPLC/MS, and HPLC/MS/MS. J Food Sci. 2008;73(5):C378-C389.
- Lorrain B, Ky I, Pechamat L, Teissedre P-L.
Evolution of analysis of polyhenols from grapes, wines, and extracts. Molecules. 2013;18(1):1076.
- Robbins RJ, Leonczak J, Li J, et al. Determination
of flavanol and procyanidin (by degree of polymerization 1-10) content of
chocolate, cocoa liquors, powder(s), and cocoa flavanol extracts by normal
phase high-performance liquid chromatography: collaborative study. J AOAC Int. 2012;95(4):1153-1160.
- Kelm MA, Versari A, Parpinello GP, Thorngate JH.
Mass spectral characterization of Uva
longanesi seed and skin extracts. Am
J Enol Vitic. 2012;63(3):402-406.
- Kuhnert S, Lehmann L, Winterhalter P. Rapid
characterisation of grape seed extracts by a novel HPLC method on a diol
stationary phase. J Funct Foods. 2015;15:225-232.
- Li W, Fong HHS, Singletary KW, Fitzloff JF.
Determination of catechins in commercial grape seed extract. J Liq Chromatogr Relat Technol. 2002;25(3):397-407.
- Ma W, Waffo-Téguo P, Jourdes M, Li H, Teissedre
P-L. First evidence of epicatechin vanillate in grape seed and red wine. Food Chem. 2018;259:304-310.
- Zhang M, Sun J, Chen P. A computational tool for
accelerated analysis of oligomeric proanthocyanidins in plants. J Food Comp Anal. 2017;56:124-133.
- Ricci A, Parpinello GP, Palma AS, et al.
Analytical profiling of food-grade extracts from grape (Vitis vinifera sp.) seeds and skins, green tea (Camellia sinensis) leaves and Limousin
oak (Quercus robur) heartwood using
MALDI-TOF-MS, ICP-MS and spectrophotometric methods. J Food Comp Anal. 2017;59:95-104.
- Hümmer W, Schreier P. Analysis of
proanthocyanidins. Mol Nutr Food Res. 2008;52(12):1381-1398.
- Yang Y, Chien M. Characterization of grape
procyanidins using high-performance liquid chromatography/mass spectrometry and
matrix-assisted laser desorption/ionization time-of-flight mass spectrometry. J Agric Food Chem. 2000;48(9):3990-3996.
- Anastasiadi M, Zira A, Magiatis P, Haroutounian
SA, Skaltsounis AL, Mikros E. 1H NMR-based metabonomics for the classification
of Greek wines according to variety, region, and vintage. Comparison with HPLC
data. J Agric Food Chem. 2009;57(23):11067-11074.
|